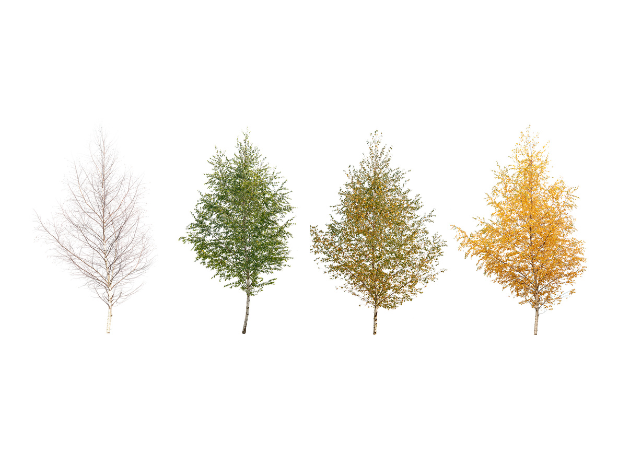
Tree in four different seasons: winter, spring, summer, fall.
How Seasonal Shifts in the Northern High Latitudes Impact Global Carbon Levels and Climate Stability
Climate change affects not only temperatures but also how ecosystems manage and cycle carbon dioxide (CO₂). Below we explore how rising temperatures and increasing CO₂ levels in Arctic and boreal regions—collectively called northern high latitudes (NHL)—are creating seasonal shifts in CO₂ levels. These changes impact our planet’s “carbon thermostat” and could intensify global warming if left unchecked. Let’s dive into the drivers behind these changes and how we can use this knowledge to shape a healthier future for our planet.
Defining Seasonal Cycle Amplitude (SCA)
Imagine Earth “breathing” with each season: in the spring and summer, trees and plants in the northern high latitudes absorb CO₂ during photosynthesis, much like an inhale. They use this CO₂ to grow, pulling it from the atmosphere and helping cool the planet. When autumn and winter arrive, these plants release CO₂ back into the air as they decompose—much like an exhale. This seasonal fluctuation in CO₂ is known as the Seasonal Cycle Amplitude (SCA).
Over the last several decades, the “inhale” in summer and “exhale” in winter has become more extreme. Plants are taking in even more CO₂ in warmer months and releasing more in cooler ones. This intensifying cycle is linked to higher CO₂ levels in the air and warming temperatures in the NHL, turning nature’s “breath” into a stronger force in the global carbon cycle.
Primary Drivers of SCA Increase
The increase in the seasonal CO₂ cycle, especially in the NHL, is due to several interacting forces. Here’s a look at the primary drivers behind this intensified “breathing”:
- Warming Temperatures: Arctic areas are warming faster than the rest of the world, which means that plants have a longer growing season to capture CO₂. This extended period of photosynthesis results in more CO₂ being absorbed during warmer months.
- CO₂ Fertilization: Plants use CO₂ as fuel to grow. With more CO₂ in the atmosphere, plants have more “food” available, which can increase their growth and further boost CO₂ absorption.
- Increased Respiration: Warmer temperatures cause more CO₂ to be released back into the atmosphere as organic matter decomposes. This process, called respiration, also happens in winter due to permafrost thaw, releasing even more CO₂.
These factors combined are driving an intensified cycle, making the NHL a more powerful influence on our planet’s CO₂ levels.
Regional Influences
Different regions within the NHL—primarily the Arctic areas of North America and Eurasia—play unique roles in this changing cycle. Here’s how each contributes:
- Eurasian Boreal Forests: These forests, especially in Siberia, are major players in absorbing CO₂. Warmer temperatures have enabled these forests to grow longer and stronger, contributing significantly to CO₂ uptake.
- North American Boreal Forests: Although North America’s boreal forests are also absorbing CO₂, they are more sensitive to drought. This means they may absorb less CO₂ during dry years compared to Eurasia’s forests, which are often moister due to atmospheric changes.
Differences in forest types, moisture levels, and permafrost also mean that these regions respond to climate change in varied ways, affecting their role in the carbon cycle.
Projections for the Future
Looking ahead, the seasonal cycle of CO₂ is expected to continue intensifying in the NHL throughout the 21st century. Under high-emission scenarios, scientists project that by the end of the century, the NHL’s seasonal CO₂ cycle could be 75% stronger than it was in the 1980s.
What does this mean for global climate? This intensified “breathing” cycle means the NHL will continue to influence Earth’s “carbon thermostat” more dramatically. With higher CO₂ intake in the growing season and increased release during the colder months, this cycle could speed up the warming effects of greenhouse gases on our climate.
Recommendations for the Future
To better understand and manage these changes, scientists recommend several strategies to improve our knowledge of the carbon cycle in the NHL and inform climate policy:
- Expand Monitoring Networks: Building more observation stations in under-monitored areas like tundras and Siberian forests will provide a clearer picture of CO₂ dynamics and seasonal trends.
- Refine Climate Models: Current models should better account for factors like permafrost thaw and snow cover to accurately predict seasonal CO₂ fluctuations.
- Support More Research: Understanding the impacts of landscape changes—such as forest growth, wildfires, and vegetation shifts—will help pinpoint how each factor influences CO₂ release and capture.
Taking these steps will help scientists and policymakers better gauge the impact of NHL ecosystems on the global carbon cycle and adapt climate policies accordingly.
Summing Up
Understanding the “breathing” cycles of the NHL offers a valuable key to shaping our climate future. By integrating more data from these regions, scientists can strengthen climate models, allowing for improved predictions and more precise climate targets. These insights also enhance policy decisions, as a better grasp of Arctic and boreal ecosystem dynamics can guide effective climate policies tailored to address the growing impact of CO₂ levels from these areas.
This seasonal “breathing” of Earth’s northern high latitudes reminds us that even the planet’s most remote areas have a crucial role in our shared climate future. By monitoring and adapting to these changes, we can contribute to a healthier, more balanced Earth.
Source: Liu, Z., Rogers, B. M., Keppel-Aleks, G., et al. (2024). Seasonal CO₂ amplitude in northern high latitudes. Nature Reviews Earth & Environment, 5(11), 802–817. https://www.umt.edu/news/2024/11/110824ntsg.php